Evolution of
Low-Mass Stars (M < 10 solar masses)
- H shell burning: H
is depleted in the core, He core shrinks; T rises around
the core, energy production by H fusion continues at a faster
rate in a shell, and the star becomes brighter.
- Star growth: Envelope expands,
so it cools down, while the core shrinks and heats up; The star becomes a red giant. (Very low-mass stars end their lives at this stage as He white dwarfs.
- Sunlike stars: When T in the core reaches 108 K, He burning to
C starts with a flash; The core expands and the luminosity decreases, as the star moves to
the horizontal branch of the HR diagram.
- He shell burning: Eventually He is depleted in the core, and the
C core shrinks; T rises, the envelope expands
> Larger red giant phase.
|
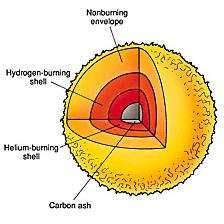 |