Gravitational waves and their detection: the ringdown phase
Gravitational waves were theoretically predicted by Einstein in 1916, soon after the
formulation of general relativity. But... do they really exist?
Unlike electromagnetic waves (verified experimentally by Hertz less than two decades after being predicted
by Maxwell), gravitational waves have not yet been experimentally detected, although there has been an indirect
verification through the observation of a binary pulsar. Hulse and Taylor
were awarded the Nobel prize in 1993 for this discovery.
|
Einstein's theory of
relativity asserts that information travels at a finite speed, and never
faster than the speed of light. Since Einstein's theory is so well-tested, it is
reasonable to adopt this as a starting point. If we extend this result to gravity, then
something must carry information
about the gravitational force. These carriers are the gravitational waves.
Gravitational waves are commonly referred as ``ripples in the fabric of spacetime'', analogous to water waves
on the surface of a lake or the ocean. In practice, this means that
gravitational waves are gravitational tidal forces traveling through space.
The animations show what happen to a ring of particles at rest when a plane
gravitational wave hits the ring perpendicularly to it.
|
|
|
Attempts to measure the direct effects of gravitational waves on Earth detectors were pioneered by Joseph
Weber, forty years ago. Those efforts continue nowadays with more modern gravitational wave detectors, such as
LIGO, GEO or VIRGO and
the planned space-based detector LISA. The reason for the difficulty in
detecting gravitational waves is that gravity is much weaker than electromagnetism. The extreme
feebleness of the waves is the major obstacle to the technological manipulation of gravity, thus the study
of gravitational radiation must rely on powerful natural sources in the universe. Unfortunately, the same
feebleness that inhibits the generation of gravitational waves also afflicts their detection, so that even a
flux of gravitational energy at the Earth's surface comparable to the heat and light of the Sun is unlikely
to be detected by present terrestrial equipment. The bright side of this feeble interaction is that the
waves will reach the detector almost uncorrupted. |
The leading candidate source of detectable waves is the inspiral and merger of pairs of stellar- and
intermediate-mass black
holes. The signal from such sources should comprise three pieces: inspiral, merger and
ringdown waveform. The inspiral waveform originates from that part of the decaying orbit leading
up to the innermost stable orbit. The nature of the merger waveform is largely unknown. The
ringdown waveform originates from the distorted final black hole, and can be considered the typical sound of black holes. A good
and accurate way to think about this stage is to imagine that the distorted black hole behaves
like a bell.
| 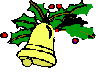 |
The ringing of a bell produces as we all know a characteristic sound. The ringing of a black hole
also produces a characteristic signal, called quasinormal ringing or ringdown. This means the black hole is
relaxing and settling into a new static phase.
|
|
The figure to the left shows the gravitational waveform for a typical inspiral and merger of compact bodies
leading to a black
hole. The waveform has three distinct phases: In the inspiral phase, the signal
is basically a sinusoid and the orbits of the bodies are well described by Newtonian dynamics. The
chirp in the signal at the end of this phase is due entirely to gravitational radiation emission: as
the system loses energy, the orbital separation shrinks and the frequency increases. In the
merger phase, the bodies are so close together that relativistic effects become important. The
orbital parameters change very quickly in this phase, corresponding to the instant of collision.
In the ringdown phase, the signal is an exponentially damped sinusoid. The features of this
signal (ringing frequency and damping time) are independent of the process that led to it.
|
Typical data coming out of the detectors will not at all look like the figure above. Real data also includes
all sort of dirty effects, called noise (see figure). Fortunately, there are well understood techniques to dig out
signals from noise, so not all is lost! This requires to know with great accuracy the form of the signal at
the source. Thus many research groups use supercomputers and sophisticated techniques to study
gravitational waves from binaries, black holes and other processes. Gravitational waves will soon be
detected!
|
|
Watch the pictures
of the Gravitational and Theory Group trip to LIGO! |
|
[Text by V. Cardoso & M. Cavaglià]